|

Oligomers
Help Us Keep Our Memories
Memory
Loss in Alzheimers Reduced for the First Time
|
|
According to the amyloid hypothesis, the amyloid plaques that form between the brain’s neurons as it ages are toxic and hence cause the cognitive losses associated with Alzheimer’s. Although this hypothesis has not remotely explained all the mysteries of Alzheimer’s,
researchers have devoted considerable effort to understanding the mechanisms by which these plaques form.
To begin with, what does the term “amyloid” mean? The answer goes back to the 19th century, when German physician Rudolf Virchow was studying a disease in which organs such as the heart, spleen, liver, and tongue swelled and hardened. To try to identify the substance responsible for this disorder, Virchow placed tissues from these organs in contact with Lugol’s solution, which contains iodine. According to the science of the day, if the tissues then changed colour, that would indicate the presence of glycogen or starch—in other words, chains of sugars.
Virchow
found that Lugol’s solution did indeed cause the tissues to change colour. He attributed this change to a starch-like substance in the tissues, which he named “amyloid”, from the Greek amylo (starch) and -oid (similar to). But a few years later, it was shown that the substance that Virchow had stained was not a starch or sugar but a protein. Other stains, such as Congo red and thioflavin, also bound to this amyloid protein material. And in 1927, Belgian psychiatrist Paul Divry found that Congo red also stained the “senile plaques” that Alois Alzheimer had described two decades earlier.
For the next several decades, scientists tried in vain to determine exactly which protein formed the central component of what were now being called amyloid plaques. It was not until 1984 that American research pathologist George Glenner
and his team characterized the structure of the protein molecules that agglutinate to form amyloid plaques. He discovered that what makes this protein “amyloid” is not the linear sequence of the amino acids that compose it, but rather its secondary structure—the way it is organized in space.
Certain
proteins, or certain peptides (which are short proteins) fold in space into special structures known as beta-pleated sheets. And it is the high content of beta-pleated sheets in the protein (peptide) that Glenner was investigating that accounts for its affinity for Congo red and thioflavin—in short, for its amyloid nature.
These beta-pleated sheets are also what makes this protein so compact, stable, and insoluble.
Because the peptide that Glenner identified had never been described previously, he named it A-beta peptide (A for amyloid and beta for the beta-pleated sheets). We now know that A-beta peptide, also commonly known as beta-amyloid,
comes from the cleavage of another, larger protein called amyloid protein precursor(APP).
APP is a transmembrane protein, which means that the APP molecule passes through the neuron’s cell membrane. APP is highly present in the central nervous system, where it is found at the synapses between neurons. APP,
like the beta-amyloid that is derived from it, is thus a normal component of the organism. For example, APP helps neurons to grow, to survive, and to repair themselves when they suffer damage.
For the beta-amyloid peptide to be released, APP
must be cleaved at two locations by two special types of enzymes.
First, beta-secretases cut the APP’s chain of amino acids at a certain distance outside the cell membrane. Then gamma-secretases make another cut, this time inside the cell membrane, thus releasing the beta-amyloid peptide.
The length of this peptide varies from 38 to
42 amino acids. The two main forms of beta-amyloid have 40 and 42 amino acids, respectively, and the latter is the one that has the greater propensity to agglutinate and thereby form amyloid plaques. |


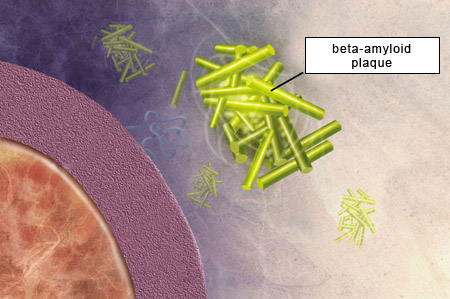
|
|
|