Tool Module:
Your "Mental Stopwatch" Scientists have
always been intrigued by the human ability to perceive the passage of time. More
specifically, they have wondered whether this ability to feel time passing was
governed by a special “clock” in the brain, or whether it was simply
a by-product of more general faculties such as memory or attention. One
thing is certain: all humans have a “mental stopwatch” that governs
our perception of time on a scale ranging from a second to a minute. This mental
stopwatch thus occupies the temporal window between the biological clock that
governs our circadian cycles, which have periods of approximately 24 hours, and
all of the oscillations associated with our brain-cell activity, which have periods
measured in milliseconds. Your mental stopwatch tells you things such as
how fast you have to run to catch a baseball, or when to snap your fingers to
keep time with a song, or how much longer you can linger in bed once your alarm
clock has gone off. This stopwatch also helps you to understand the chronological
sequence of events. To understand what someone is saying in a conversation, for
example, your brain must recognize the duration of the vowel and consonant sounds
you are hearing, mentally organize them into words and sentences, perceive the
overall structure of the thoughts they are expressing, and generate a sensible
reply at the right time. Here’s another familiar example. When a
traffic light turns yellow as you are driving toward an intersection, you maintain
a continuous mental estimate of how long it has been yellow, while comparing this
value with your memory of how long yellow lights last. Finally, as you reach the
intersection, you decide how much time you have left before the light turns red,
and you act accordingly. As you can readily see, it would be hard to think
of any complex human behavioural process in which this mental stopwatch is not
involved. *** The operation of this stopwatch must
of course be linked with several other faculties of the brain, such as attention,
which lets you note the start and end of time intervals, and memory, which lets
you store their duration for future comparisons. Your subjective perception
of time passing is closely connected to how much attention you are paying to the
time interval in question. If you find the material that you are reading right
now intriguing, then the time you spend reading it will seem short. But if you
find it boring, then the time will seem long. You will then take this duration,
as you have perceived it subjectively, and transfer it into your working memory.
There you can maintain and manipulate your mental representation of this duration
for a certain time—for instance, the time it takes to compare with another
duration that you have estimated recently, or yet another that you stored in your
long-term memory long ago. The location of the brain circuits that constitute
the physical substrate of this mental stopwatch is still much debated. The basal
ganglia and the cerebellum have long been considered
prime candidates, because damage to these parts of the brain disrupts behaviours
that are necessary for precise calculations of time. But because these abnormal
behaviours can also be attributed to more general disturbances of the motor system,
the contribution of each of these structures to our ability to perceive durations
remains ambiguous. It has also been hypothesized that the activation of
the basal ganglia may occur earlier in this process, for the purpose of encoding
time intervals, but that the activation of the cerebellum may occur later, which
implies that it is involved in something more than just estimating durations.
Other studies of brain lesions in animals and humans have shown that the
frontal and parietal lobes also may be involved in the stopwatch function, but
indirectly, through their role in attention and working memory. Individuals
who have suffered right-hemisphere lesions also often have more trouble in estimating
durations. This same tendency has been seen in brain-imaging studies in which
the right hemisphere has been found to make the greater contribution to duration-comparison
tasks. *** The reason that the mechanism behind the
mental-stopwatch function is so hard to understand is that it does not seem to
be located in any single area of the brain. Thus it contrasts with our central
biological clock, which is located in the suprachiasmatic nuclei, receives definite
inputs from the visual system, and triggers circadian behaviours and the cyclical
secretion of hormones. As scientists have acquired more
and more data about the anatomy and functioning of the brain, they have proposed
various models to try to explain the mechanism by which the mental stopwatch works.
The loop circuits connecting the cortex to the basal ganglia thus inspired a model
in which the computations of duration were based on the time that a nerve impulse
took to make a trip through this loop. In this model, the mental stopwatch was
a sort of biological simulator that independently generated a “tick-tock”
every time a cycle through this loop was completed. But this model, which
treats duration estimates as something that the brain calculates independently,
then adds to our other mental processes, has now been set aside in favour of other
models in which the calculation of duration is intrinsically linked to the basic
information coming from our sensory inputs. For example, researchers such as Warren
H. Meck, of Duke University in North Carolina, have developed a new theory of
the mental stopwatch, based on the detection of coinciding oscillations in neural
activity. Functional magnetic resonance imaging (fMRI) has played a major
role in this research, by letting scientists more accurately observe the brain
structures involved in estimating intervals and the chronological sequence in
which these structures are activated. Through fMRI, it has been shown that when
the brain performs an interval-estimation task, it is the basal ganglia that are
activated first, and in particular the striatum, which has a
population of richly interconnected neurons that receive signals from many other
parts of the brain. The dendrites of these neurons are covered with 10 000
to 30 000 dendritic spines, each of which receives information from a different
neuron located in another area of the brain. The striatum is one of the very few
places in the brain where there is such a convergence of thousands of neurons
on single neurons. The “coincidence detection” model proposed
by Meck and other researchers starts from the fact that the neurons of the cortex
have highly varied rhythmic activities. Many of these neurons fire 10 to 40 times
per second spontaneously, without receiving any external stimulus. Each of these
cortical neurons sends signals to the dendrites of the cells of the striatum,
which integrates this special “neuronal music ”. But when a specific
event occurs—for instance, when a traffic light changes from green to yellow—the
cortical neurons fire simultaneously. This produces a characteristic “evoked
potential ”some 300 milliseconds later. This evoked potential acts somewhat
like the firing of a starter’s pistol, after which the cortical neurons
resume their usual disorderly oscillatory activity. But because all of
these cortical neurons have now been put into phase by the event in question,
their resumption of their natural oscillation frequencies causes a typical, reproducible
pattern in the striatal neurons onto which they converge. The shape of this pattern
varies with the passage of time. According to Meck’s mode, it is this singular
pattern of the time that passes after a particular event that the striatal neurons
use to measure the elapsed time. To remember the duration of an interval,
such as the amount of time that that traffic light stayed yellow, another brain
structure would then come into play: the substantia nigra, which
is often associated with the basal ganglia. According to this model, following
the event that defined the end of the interval—in our example, when the
traffic light changed from yellow to red—the substantia nigra would send
out a discharge of the neurotransmitter dopamine. This sudden influx of dopamine
would enable the striatal neurons to remember the oscillation pattern that they
received at that precise moment, somewhat like a photo that they could subsequently
use to identify similar patterns and hence equivalent intervals. For indeed, that
is exactly what this model postulates: that every instant after the start of an
interval has its own distinct signature. When a striatal neuron has thus
learned the oscillatory signature associated with the duration of a particular
event, something different will happen the next time this event occurs: a discharge
of dopamine from the substantia nigra at the start, rather than the end, of the
cortical neurons’ evoked potential associated with the start of the event.
This influx of dopamine would tell the striatal neurons to start analyzing the
oscillatory patterns that they were receiving and to continue analyzing them until
they recognized the pattern corresponding to the end of the event interval. At
that precise moment, a signal would be sent from the striatum to the thalamus,
which communicates with the cortex and the so-called higher functions
such as memory and decision-making. Thus the signal would return to the cortex
after passing through the striatum, but this time the brain would know how long
it has left before the event ends. So, to return to our traffic-light example,
you can then decide whether to drive through the intersection or to apply your
brakes. 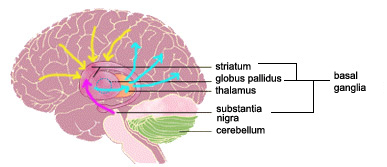
The
hypothesized role of dopamine in this mental-stopwatch model
is supported by experiments that have been done with individuals who produce less
dopamine, such as people with Parkinson’s disease. When these people are
asked to estimate the length of time intervals, they invariably underestimate
them—in other words, for these people, the time has seemed to pass more
quickly. When they are treated with medications that raise the dopamine levels
in their brains, their performance improves at least somewhat. People’s
dopamine levels also tend to decline as they grow older, starting in their twenties,
which may explain why time seems to go faster as you grow older. The opposite
phenomenon also seems to occur: substances such as cocaine increase the availability
of dopamine and speed up the mental stopwatch, so that time seems to pass more
slowly. Epinephrine and other stress hormones also speed up the mental stopwatch,
which may explain why a few seconds can seem interminable when you are having
an unpleasant experience. Lastly, states of intense concentration or intense
emotions may overwhelm the mental stopwatch or simply bypass it, thus creating
moments where time seems to be suspended or not to exist at all. As musicians
and athletes know, there are ways of training this mental stopwatch to make it
more accurate. The average person’s mental stopwatch estimates time intervals
with an accuracy ranging anywhere from 5 to 60 % of their actual length, and the
margin of error grows with the length of the interval. That is why people still
find it so handy to wear an actual watch on their wrist, or to carry a cell phone
that displays the time. Based on: “Times of Our
Lives”, by Karen Wright, Scientific American, September 2002
|