|
In addition to the biological
clock, the brain contains another time-dedicated component
that works more like a stopwatch. Instead of providing an
absolute time reference the way a clock does, this “mental
stopwatch”lets you estimate how much time
has elapsed since a given event. For example, suppose a traffic
light changes from green to yellow as you are approaching
an intersection in your car. When you actually reach the
intersection, your decision whether to drive through it or
not depends on how much time has elapsed since the light
turned yellow.
This mental stopwatch that lets you
monitor the passing of time appears to involve the cortex,
the thalamus, and another structure that seems to play a
central role in this process: the
striatum of the basal ganglia. |
|
|
The first gene identified as being
involved in the circadian cycle of a living organism was the period gene,
in the fruit fly, in 1971 (follow the History Module link to
the left). The second was the clock gene, identified
in mice in 1997. Since then, scientists have learned a great
deal about the molecular mechanisms of the biological clocks
of various species.
One of the first things scientists learned was that all of these
mechanisms are based on negative feedback loops (follow the Tool
module link to the left), in which proteins
in the cell’s cytoplasm enter its nucleus, where they inhibit
their own production. In mammals, for example, the period (per)
and cryptochrome (cry) genes are activated by a
complex of the proteins CLOCK and BMAL1. The DNA of these genes
is then transcribed into messenger RNA, which is translated into
the proteins PER and CRY in the cytoplasm. These proteins then
form complexes (PER/CRY and PER/PER) that enter the cell nucleus
and interact with the CLOCK/BMAL1 complex so as to inhibit the
transcription process and hence the production of PER and CRY.
We also now know that several of the genes involved in the biological
clock are well preserved in evolutionary terms and occur in many
species. Also, several types of a given gene sometimes occur in
the same species, such as the three types of period gene
(per1, per2, and per3) and the two types
of cryptochrome gene (cry1 and cry2)
in the neurons of the suprachiasmatic
nuclei in humans.
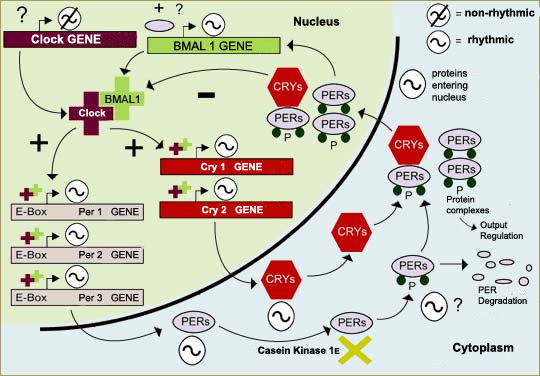
Adapted from: Whitmore,
D. et al.: A Clockwork Organ. Biological Chemistry 381,
793-800 (2000)
Lastly, we know that this complex feedback
loop is subject to the influence of the ambient light, which causes
it to be synchronized with the cycle of day and night. When a light
stimulus alters a photosensitive molecule (see box below), the
production of PER 1 and PER 2 in the SCNs increases, which in turn
induces changes in the progression of the loop.
But
knowing the main internal mechanisms of the biological clock
solves only half the problem, because this clock co-ordinates
many functions, such as sleep, body temperature, and the secretion
of various hormones. The other half of the problem, which is
the subject of a large share of the chronobiological research
going on today, is the output: in other words, how does
the body’s biological clock speak to all these other systems?
In some cases, this communication might
involve a direct interaction between the components of the clock
and the gene for a particular hormone. For example, the proteins
CLOCK and BMAL1 bind to the E-box element not only on the per gene
but also on the gene for vasopressin. Like the production of
the protein PER, the production of vasopressin will be interrupted
when a sufficient number of PER molecules have entered the cell
nucleus and bound to the CLOCK/BMAL1 complex, thus deactivating
the production of mRNA not only for PER but for vasopressin as
well.
The rate of production of a hormone may therefore
fluctuate over a 24-hour cycle because of this kind of close linkage
with the components of the body’s biological clock.

How is this complex
feedback loop influenced by light so as to be synchronized
with the day/night cycle? Scientists agree that the first
link in the process by which mammals reset their biological
clock each day to keep it in phase with this cycle must be
a photopigment that converts light energy into neuroelectric
impulses. But since about the year 2000, the identity of
this photopigment has occasioned much debate. One camp of
scientists argues that it is melanopsin, while
the other champions cryptochrome.
As happens so often in these pitched battles between scientists,
both sides can marshall data that seem to prove their case,
which is what makes the argument so fascinating.
Cryptochromes
were first discovered in plant cells, where the proteins
CRY1 and CRY2 trigger growth in response to blue
and ultraviolet light (follow the Tool module link
to the left). Subsequently, cryptochrome was
shown also to be a key component in the feedback
loop of the mammalian biological clock.
In fruit flies, crytpochrome
acts as a photosensitive pigment that reinitializes
the insect’s biological clock. Thus it is this
protein that enables fruit flies to adapt their circadian
cycles in experiments that reproduce the effects of jet
lag. |
Hypothetical Structure
of the Protein Cryptochrome
Source: The Zhong Group, Ohio State
University |
But in mammals, according to some researchers,
cryptochrome has permanently lost this photoreceptive function
and has become just one component in a molecular clockwork
mechanism whose activity no longer depends on light. This
view is supported by experiments showing that when flies
are deprived of all their opsins and cryptochrome, they can
no longer synchronize their circadian cycles, but when mice
are deprived of their rods, cones, and cryptochromes, they
still retain a residual response to light.
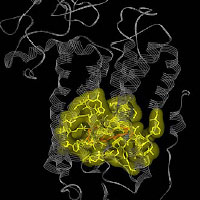
Hypothetical
Structure of the Protein Melanopsin in
Djungarian Hamsters
Source: Dr. Alexander Lerchl
|
These researchers instead identify
melanopsin as the photopigment that entrains the mammalian
biological clock. Melanopsin is found in a very small
percentage (about 1%) of the retinal ganglion cells
that innervate the suprachiasmatic
nuclei. These same ganglion cells that contain
melanopsin also innervate other parts of the brain
that are affected by light intensity, such as the brain
cells involved in the pupillary response.
When the genes both for opsins in
the rods and cones and for melanopsin are “knocked
out” (eliminated), mice become completely insensitive
to the length of day and night. But if only the melanopsin
genes are “knocked out”, then only a modest
reduction in the circadian response to light is observed.
These observations have formed the basis for a model
in which opsins and melanopsin are posited to play
necessary and sufficient but redundant roles in circadian
photoreception in mammals. |
But it’s not that simple, because
other observations do support a role for cryptochrome in
mammalian photoreception. For example, the pupillary response
to blue light is 20 times less sensitive in mice that have
no rods, no cones, and no cryptochrome than in mice that
have no rods and no cones but do have cryptochrome. Further
evidence: when mice are deprived of all sources of Vitamin
A, they cannot form retinaldehyde, the essential co-factor
for all opsins. But though these mice are blind, and their
pupillary response is about 1/10 000 that of normal
mice, their ability to transmit light signals from the retina
to the suprachiasmatic nuclei seems relatively unimpaired.
Contrary to the results obtained with
mice whose genes for opsin have been deactivated and that
show no response to light, the experiments with mice that
are deprived of Vitamin A paradoxically seem to show that
some form of phototransduction persists. Some researchers
argue that this residual phototransduction may therefore
be attributable to cryptochromes.
To reconcile these apparently contradictory
findings, a new model may be needed, in which cryptochromes
and melanopsin work together to generate the retinohypothalamic
signal. But that will probably have to wait until scientists
know more about this subject, and in particular about the
cascade of biochemical reactions involving cryptochromes. |
|
|