|
Experiments on the
regulation of wakefulness have shown that this function,
which is essential for survival, involves several redundant
structures. But rather than consisting of well defined “centres”,
these wakefulness systems actually operate in networks.
In other words, when one of these systems is excited pharmacologically,
all of the others are generally activated in turn.
Likewise, when any one of these
systems is deactivated, wakefulness returns almost completely
within a few days. Thus, none of these structures, taken
in isolation, is indispensable for the activation of
the cortex. |
| |
THE
BRAIN DURING REM SLEEP |
|
The amygdala is
one of the parts of the brain that is most active during REM
sleep, but this state is actually generated deep in the brainstem.
However, the
set of cortical and limbic structures involved in REM sleep do
not just passively submit to orders issued by the brainstem.
On the contrary, the particular kind of dream images associated
with REM sleep are the result of a dynamic interaction between
certain key structures in the brainstem and the rest of the brain.
The discovery that REM sleep occurs in almost all mammals opened
the door to the first animal experiments with REM sleep, which
were conducted by French neurobiologist Michel Jouvet in the
early 1960s. By studying the effects of lesions and electrical
stimuli in the brain, particularly in cats, Jouvet was gradually
able to home in on the brainstem as the location of the
neurons that trigger REM sleep. One of Jouvet’s
methods involved dividing the brain into two halves and trying
to see which of them maintained the function in question. He
then divided the “winning”half and repeated this
process to further narrow down the brain area involved in this
function, and so on until he had isolated certain essential nuclei.
Because each part of the brain is usually
densely interconnected with the others, one might expect that
the slightest subdividing of the brain would eliminate the electrical
activity specific to dreaming. But surprisingly, the parts of
the brainstem that generate REM sleep can continue to do so even
if they are disconnected from practically all of the rest of
the brain. In contrast, the destruction of even a small portion
of the brainstem itself can prevent the expression of REM sleep.
What then are these nuclei that Jouvet found in a very small
portion of the brainstem and that are both necessary and sufficient
for the neurological activity of REM sleep? They are located
essentially in the pons, and in particular in its rostral portion,
where it meets the midbrain.
One of these nuclei that are essential for REM sleep is the nucleus reticularis
pontis oralis (RPO), which extends from the caudal
midbrain to the rostral pons. A population of neurons in this
area is selectively active during REM sleep. Lesion studies
of this small area of the pontine tegmentum have also shown
that it is required in order for normal REM sleep to occur.
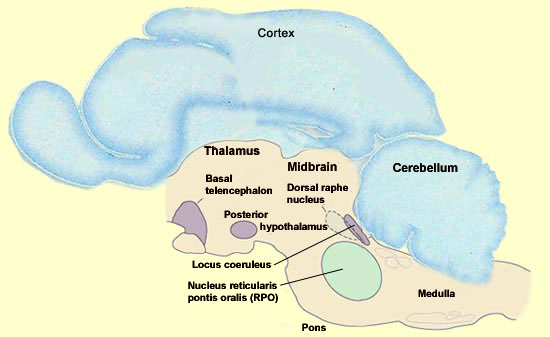
Sagittal section of the
brain of a cat, the model of choice for studying REM sleep,
with a simplified diagram of certain structures involved in
controlling REM sleep (Adapted from Principles of
Neural Science, Kandel,
Schwartz, and Jessell, Eds., Appleton & Lange, Pub.)
REM sleep can be induced by the microinjection
of acetylcholine agonists into
this small area. It is not known whether this area is sufficient
to generate all of the aspects of REM sleep, for example, the
way the suprachiasmatic nucleus suffices to maintain all aspects
of the circadian cycle.
That a sudden elevation in the activity of the cholinergic
neurons of the pons is necessary for the onset of REM sleep
may seem somewhat strange, because the brain’s acetylcholine
systems are known for their role in wakefulness.How then
can this system associated with wakefulness be activated during
one of the phases of sleep? The answer probably lies in the
simultaneous reduction in the activity of two other nuclei
that produce other wakefulness neurotransmitters, a reduction
that is just as necessary for REM sleep as the increase in
cholinergic activity. The two nuclei in question are the dorsal
raphe nucleus, a group of serotonergic neurons, and
the locus coeruleus, a group of noradrenergic
neurons, and both of them are located in the rostral portion
of the pons.
REM sleep cannot begin unless all activity in these two main
aminergic systems of the brainstem ceases. The serotonergic and
noradrenergic neurons in these systems are referred to as REM-off neurons
because of their inactivity during REM sleep, and they can be
said to act as a sort of permissive system for REM sleep, in
the sense that REM sleep becomes possible only when their activity
has ceased. The shutdown of these neurons might, for example,
help to suppress consciousness during REM sleep. Also, the periods
of REM sleep end when these aminergic neurons become active again.
One of the most singular characteristics of REM sleep—the
total paralysis of the body that it causes —also
is explained by phenomena that occur in certain parts of the
brainstem.
| |